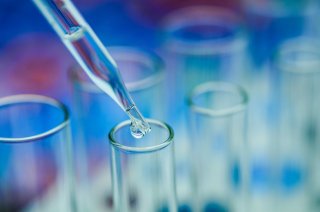
Longread
Synthetic biology
Medicine-producing bacteria, lab-built cells, synthetic vaccines and an electric nose: synthetic biology opens up a whole new world for scientists. This branch of science – a combination of biology, physics, chemistry and information technology – makes it possible for us to make things that we could previously only dream of. Sometimes, it is not far removed from science fiction. We at Wageningen University & Research (WUR) use synthetic biology to produce more and better food, improve health and create a greener environment.
What is synthetic biology?
Synthetic biology is nature-based design. 'Rather than being a branch of biology, like ecology or medical biology, it's much more a way of working,' explains Vitor Martins dos Santos. He is the head of WUR's Synthetic Biology department. 'Synthetic biology has similarities with industrial design. Engineers design a new car by improving all sorts of individual components (engine, chassis, wheels, bodywork) and combining them into better cars, and in synthetic biology we combine knowledge of proteins, DNA, micro-organisms and cells into new applications.' At first glance, synthetic biology has much in common with biotechnology. 'The difference is that the purpose of biotechnology is to improve existing life forms. In synthetic biology we take this a step further: instead of looking for new applications for existing organisms, we design new, synthetic life forms.'
- Unfortunately, your cookie settings do not allow videos to be displayed. - check your settings
Interdisciplinary approach
The development of greatly improved computers and computer models in recent years has given synthetic biology a powerful boost. Much more is now known about the structure and behaviour of organisms and molecules that occur in them. There are databases containing information about vast numbers of bacteria: what they live on, which substances they convert and how they are structured. There are also such databases for proteins and genes. And the fact that scientists are now able to isolate the genes of one organism and place them in another allows them to give bacteria an extra gene so that they produce extra substances. This enables scientists to use the knowledge in the databases to combine and simulate all sorts of things.
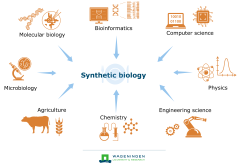
Model-driven
That makes the approach taken in synthetic biology something completely different. 'What we do actually amounts to model-driven design,' explains Vitor Martins dos Santos. 'This is completely new to biology. We usually take a problem and try to find a solution to it, focusing specifically on that particular problem.' Up until now a lot has been discovered by chance in biology. A scientist studying how moulds work discovers that a particular substance kills bacteria. And that's what ultimately led to the discovery of penicillin as an antibiotic. 'Synthetic biology leaves much less to chance. We look very specifically for models that work for various aspects of biology. That involves finding ways of standardising the methods.'
Together with stakeholders
Synthetic biology can have an impact on society: it presents ways of improving health, solving the global food problem and developing sustainability. Synthetic biology has endless possibilities. But it takes time and money. Priorities have to be set. For that purpose research scientists at Wageningen University & Research regularly talk with the people who will be working with the new products. What does society need? What should be prioritised?
Like other areas of biotechnology, synthetic biology gives rise to a number of questions. Synthetic biology makes use of genetically modified organisms and synthetic life forms, and that presents us with ethical and social issues. We discuss these with our stakeholders and always ask ourselves: is this really what we want? How far can you go with modifying organisms? Is it safe to develop synthetic organs? There are also safety issues: if you modify a population of organisms (e.g. mosquitoes that are no longer able to carry malaria), how does this affect the ecosystem in the long term? And what if our knowledge falls into the wrong hands? If we can make synthetic viruses to use as vaccines, what's to stop people with malicious intent from using the same knowledge to create pathogenic viruses? Who's in charge? Economic issues are also involved: who owns the newly developed life forms and substances? Who can earn from them?
Modifying organisms
Synthetic biology can be applied at various levels. Bacteria often have a role to play in this because we know a lot about them; they are easy to genetically modify and can multiply quickly. All bacteria have their own specific properties, which have been clearly identified in databases. The one type of bacteria is good at producing nitrogen, for instance, whereas the other affects a plant's hormone production. Another one is good at breaking down the nutrients in our food and producing vitamin K in the intestines. That diversity and comprehensive knowledge presents us with all sorts of opportunities: for our food production, our health and our environment.
Stimulating plant growth
Theo van der Lee, for example, is working with his colleagues on a way of developing the ideal microbial community for plants. 'Bacteria contained in moulds in the soil can strongly affect plant growth. They produce chemicals such as nitrogen and phosphorus from the soil, prevent certain plant diseases, influence the plant's hormone production and promote its growth. 'Each environment and each plant needs a different composition of micro-organisms in the soil. The challenge is to find the perfect composition in a given environment.' Synthetic biology could play a major role in this area, too. 'Our previous research has already established which components such a microbial community needs. We can identify the properties of micro-organisms by analysing the genome. We're now working on a model-driven approach that will make it fairly simple to come up with the ideal microbial community for each environment and plant.'
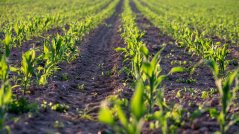
Medicine-producing bacteria
We can also use bacteria to produce medicines. The idea is simple: find or build a gene that codes for the substance you need, build this gene into a bacteria and leave the bacteria to multiply. That creates vast quantities of bacteria that can produce the substance you need. This might sound a bit like genetic modification. But it's completely different, explains Vitor Martins dos Santos. 'Genetic modification still involves a lot of trial and error: hoping that you take out the right gene, integrate it correctly, and, ultimately, hoping that it does what you want it to. That's in sharp contrast to how an engineer works: we start by designing the DNA on the computer before working with it in the lab. This has been made possible by all the knowledge we have of proteins and genes.'
Bacteria for diagnosing tropical diseases
A team of students – the iGEMteam 2017 – is using all the available knowledge of bacteria, genes that promote processes in bacteria, and chemical reactions for an entirely new and challenging project: making diagnostic tests for tropical diseases with the aid of bacteria. The bacteria are armed with a type of antibody known as an affibody. These small molecules react highly specifically to a single type of disease and are easy to modify. Theoretically, an affibody can be developed for any infectious disease. The bacteria are also given a detection system. If an affibody binds to a pathogen, the detection system responds by transmitting a kind of light signal that can be measured with UV light. The bacteria are kept in a device called the Mantis. This makes it a simple matter to diagnose a pathogen: all we need to do is add a drop of blood or urine to the bacteria. The device measures the UV light and displays the result on a screen.
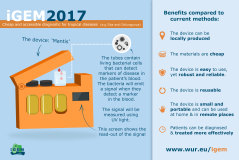
The Mantis has a number of advantages over the current diagnostic tests for tropical diseases. While being cheaper, more specific, and more precise than the current tests. It can be relatively easily adapted to new pathogens, which means that an effective diagnostic test can be quickly developed if a new disease emerges.
Pollution-detecting bacteria
Bacteria can identify other substances, such as gases, in the same way. 'This works a bit like a nose,' explains Martins dos Santos. 'A nose contains olfactory receptors, each of which reacts to a certain type of substance. It's also possible to develop bacteria that react to a certain smell and then produce an artificial odour.' There are even bacteria that can detect TNT and turn red if any of this explosive substance is in the area. This means we can locate the TNT from a distance; we don't need to go anywhere near it. In this project, too, scientists are looking for a model that can be easily adapted to any substance.
Creating new life forms
Synthetic biology, however, goes beyond modifying life forms: it's also about developing new ones. This involves building viruses, cells and organs in the lab that can be adapted to the required properties. That's something else we're working on every day.
Synthetic viruses as vaccines
We've already been using viruses to improve our health for many years, in the form of vaccines. We can modify a pathogen, such as the chickenpox virus, so that it's no longer harmful to our health but is still recognised by our immune system. This enables us to develop immunity and avoid falling ill if infected by the real virus.
- Unfortunately, your cookie settings do not allow videos to be displayed. - check your settings
These days we're taking this a step further, explains research scientist Jeroen Kortekaas. 'What we know about the structure of virus particles and the ingenious way viruses infect cells enables us to develop virus-like particles with precisely the properties we want.' This is fairly easy to do with viruses because all they actually consist of is a piece of genetic material in a globular protein. 'We build the genetic material ourselves so that it possesses precisely the right properties, and we decide which protein to put around it. This opens up all sorts of possibilities, not just for producing vaccines, but also for research. 'We can cause a virus to light up if it gets into a cell, for example. That enables us to analyse the immune system: how good is it at fighting the virus? How well is the body able to prevent the virus from getting into a cell?'
A synthetic cell
'The ultimate challenge is to build a whole cell. Not a simple virus, but a cell like those that make up people and animals,' says Vitor Martins dos Santos. A major project in which Wageningen University & Research is taking part got underway in the autumn of 2017. The aim is to build a fully functional cell from scratch. The BaSyC (Building a Synthetic Cell) project involves a partnership between several institutes in the Netherlands, including Wageningen University & Research, TU Delft, Groningen University, VU Amsterdam, Radboud University Nijmegen and AMOLF.
The main purpose of the project is to add to the body of knowledge. Much is already known about what individual parts of the cell do, but we don't yet understand how they work together to enable life. That knowledge is needed to build a cell. The developmental process is characteristic of synthetic biology: the research scientists use the current knowledge of all sorts of different cells of different organisms and use it to build a new cell. This isn't a copy of an existing organism, but a unique cell in which the properties of various life forms are combined into a new one.
Successfully building a new cell could open up many possibilities: we may be able to build new, smart and environmentally friendly materials in the high-tech industry, and produce new sustainable fuels and biologically degradable plastics. There will also be new ways of designing sustainable, safe and healthy food.
Gut-on-a-chip
Synthetic biology could also be useful to the study of how organs function. Hans Bouwmeester and Maarten Jongsma are working – each in their own way – on making what is known as a 'gut-on-a-chip'. The intestines are fairly complex because they involve more than intestinal cells, blood and antibodies. The bacteria in the intestines also play a prominent role in intestinal functions. We can use intestinal cells alone to study how the intestines react to certain substances. A complete intestine is needed for this type of study.
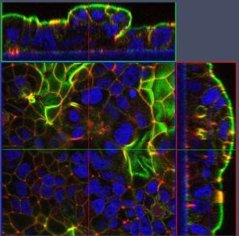
That's why Hans Bouwmeester and his team are working together with research scientists in Twente on a gut-on-a-chip: a synthetic model of the intestines – complete with blood cells, antibodies, intestinal cells and intestinal flora – on a plastic dish. They simulate all the building blocks of the real intestines where liquid flow takes place. That will enable us to study the interaction in the intestines between nutrients, micro-organisms, intestinal cells and their effects on the immune system. This helps us to accurately record the positive and negative effects of substances. Maarten Jongsma specialises in the live detection of those cells' responses to various treatments. Immune responses and satiation hormones made visible immediately.
Synthetic biology games
Would you like to experience synthetic biology for yourself? No problem! The company Biofaction has developed some games, based on real research projects, that enable you to work as a synthetic biologist:
SynMod
In this game you develop a new antibiotic to take out pathogens.
- View the game trailer here.
- The game itself is available as an app from the App Store and Google Play.
Berrymaker Game
In this game you're the owner of a start-up producing soft drinks made using synthetic biology. Make your company a success by producing the best-selling drink, taking account of prices and market demand.